Quantum Sensing


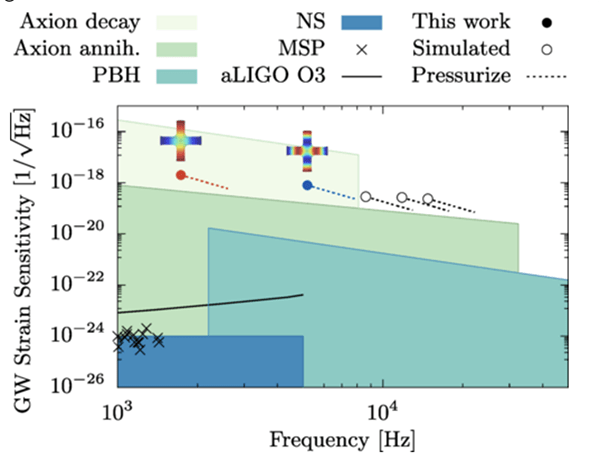
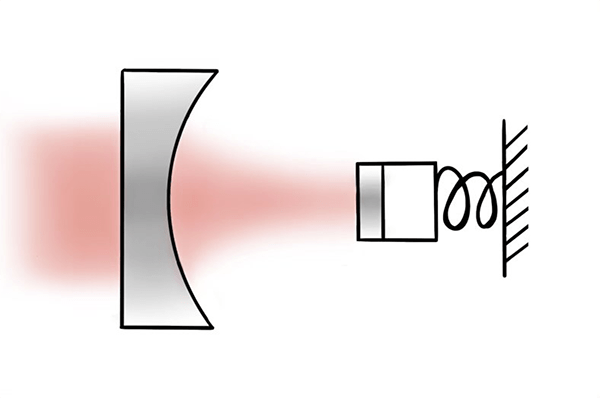
Quantum sensors leverage coherent superposition, entanglement, and quantum control to enable significant improvements in sensitivity to weak forces and fields. Such sensors have been critical to industry, healthcare, military applications, and scientific explorations. Our efforts to develop and expand the foundations of quantum sensors build on our expertise in AMO and condensed matter physics, particularly ultracold atoms, trapped ions, optomechanical systems, defect center qubits and photonics. See, for example, our portal providing high-precision atomic data.
The development of quantum technologies enables to probe many current and persisting questions about the nature of our Universe:
- What is the nature of dark matter? What is the nature of dark energy?
- Are there new fundamental interactions? Are there particles beyond those of the standard model?
- Is there a limit on macroscopic quantum superpositions?
- What is the source of matter-antimatter asymmetry in the Universe?
- How to connect gravity and quantum mechanics?
- Does general relativity hold in extreme regimes?
- Are fundamental constants actually constant?
- Are there violations of fundamental symmetries, such as CPT (charge, parity , time), permutation symmetry for identical particles, the spin-statistics connection, and Lorentz invariance?
On the application side, quantum sensing provides urgently needed capabilities across a wide range of fields:
- Study and diagnostics of novel materials and devices on the mesoscopic and nanoscale
- Highly sensitive nuclear magnetic resonance imaging for chemistry and biology
- Molecular detection for disease diagnosis
- Non-invasive detection of bioelectrical signal (e.g. neuronal and cardiovascular activity)
- Imaging of paleomagnetism for geo-science
- GPS-free navigation
Our team has expertise in tests of fundamental symmetries, development of ultra-precise optical clocks and their application to search for variation of fundamental constants, testing the Lorentz invariance, and dark matter searches as well as application of mechanical systems as detectors of gravitational waves or dark matter. Our team also works to develop spin-based quantum sensors in diamond and van der Waals (vdW) materials (e.g. hexagonal boron nitride) and apply them to probe local magnetic fields of 2D magnets and other exotic nanoscale materials. Magnetometry sensing techniques include using nitrogen-vacancies; single spin systems in van der Waals (vdW) materials; manipulating magnetic materials; theoretical quantum optics; and quantum transport.
Participating Faculty: Safronova, Singh, Ku, Chakraborty, Xiao, Jungfleisch, Ni, and Nikolic